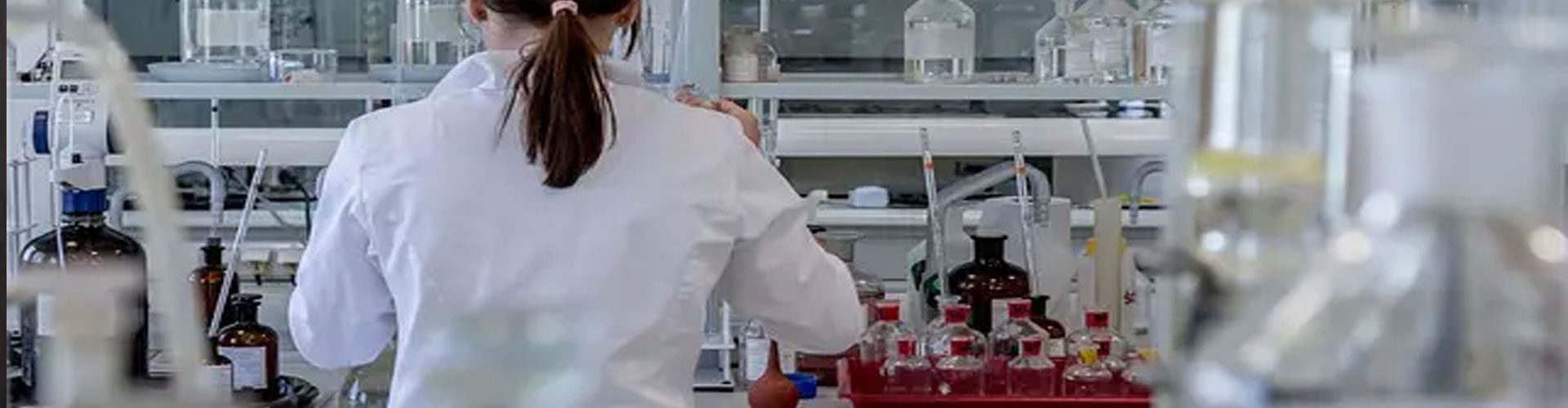
Dr. William H. Hersh
ProfessorDepartment of Chemistry & Biochemistry
Research
1. Chiral phosphites and disulfides – connections to oligonucleotide synthesis (papers A1-7)
Starting with N-sulfonyl amino acids, we showed that P-chiral trivalent phosphorus compounds such as 1 were readily accessible, and that they do not undergo inversion at phosphorus (A1, A2, A3, A7). The inversion barrier in phosphite triesters similarly appears to be high, as evidenced by sulfurization of chiral phosphites during the synthesis of chiral phosphorothioates for antisense oligonucleotides, where a sulfur atom replaces an oxygen on the phosphodiester backbone. We showed that the barrier is virtually the same as that for phosphines, with inversion occurring slowly at 150 °C, as shown for the equilibrium between 2 and 3 (A4). We synthesized chiral disulfides (for instance 4 and 5) as a potential route to chiral sulfurization of phosphorus for chiral phosphorothioate synthesis (A5, A6).
We recently found the novel route from H-phosphonodiamidite 6 and acid chlorides to give acylphosphonites such as 7, and coupling to give acyl dinucleosides such as 8. While 8 was expected to epimerize readily by analogy to acyl phosphines, it does not, and the calculated inversion barrier is over 40 kcal/mol (A8).
We recently found the novel route from H-phosphonodiamidite 6 and acid chlorides to give acylphosphonites such as 7, and coupling to give acyl dinucleosides such as 8. While 8 was expected to epimerize readily by analogy to acyl phosphines, it does not, and the calculated inversion barrier is over 40 kcal/mol (A8).
(A8)
Hersh, W. H., “Synthesis of dinucleoside acylphosphonites by phosphonodiamidite chemistry and investigation of phosphorus epimerization,” Beilstein J.Org. Chem. 2015, 11, 184-191. (Thematic Issue on Nucleic Acid Chemistry)
(A7)
Hersh, W. H.; Lam, S. T.; Moskovic, D. J.; Panagiotakis, A. J. “A Non-Karplus Effect: Evidence from Phosphorus Heterocycles and DFT Calculations of the Dependence of Vicinal Phosphorus-Hydrogen NMR Coupling Constants on Lone-Pair Conformation,” J. Org. Chem. 2012, 77, 4968-4979.
(A6)
Mukhlall, J. A.; Hersh, W. H. “Sulfurization of Dinucleoside Phosphite Triesters with Chiral Disulfides,” Nucleosides, Nucleotides & Nucleic Acids 2011, 30, 706-725.
(A5)
Mukhlall, J. A.; Noll, B. C.; Hersh, W. H. “Synthesis of Chiral Disulfides: Potential Reagents for Enantioselective Sulfurization,” J. Sulfur Chem. 2011, 32, 199-212.
(A4)
Mukhlall, J. A.; Hersh, W. H. “Measurement of the Barrier to Inversion of Configuration in Acyclic Phosphite Triesters,” Inorg. Chim. Acta 2011, 369, 62-70. (special R. G. Bergman issue)
(A3)
Hersh, W. H.; Klein, L.; Todaro, L. J. “Stereoselective Synthesis of P-Chiral Phosphorus Compounds from N-tert-Butoxycarbonyl Amino Acids,” J. Org. Chem. 2004, 69, 7355-7358.
(A2)
Hersh, W. H.; Xu, P.; Simpson, C. K.; Grob, J.; Bickford, B.; Hamdani, M. S.; T., W.; Rheingold, A. L. “Synthesis and Structural Characterization of Trivalent Amino Acid Derived Chiral Phosphorus Compounds,” J. Org. Chem. 2004, 69, 2153-2163.
(A1)
Hersh, W. H.; Xu, P.; Simpson, C. K.; Wood, T.; Rheingold, A. L. “A Chiral N-Sulfonylphosphoramide: Synthesis and X-Ray Crystal Structure of a 1,3,2-oxazaphospholidin-5-one, a Trivalent Electron-Withdrawing Amino Acid-Derived Phosphorus Compound, and Synthesis of Its W(CO)5 Adduct.,” Inorg. Chem. 1998, 37, 384-385.
2. 31P NMR and spin-spin coupling – experiment and theory (papers A7,B1-3).
We have a long-standing interest in unusual NMR spectra, such as that seen in anion “spinning” in phosphine-tungsten complexes where all six fluorine atoms of SbF6– couple to phosphorus (B2), and more recently in the observation of vastly different vicinal phosphorus-hydrogen coupling constants as a function of lone-pair conformation as shown for calculations on CH3OPH2 below (A7). We use Gaussian DFT calculations to minimize structures, analyze chemical shifts and coupling constants, and currently to develop a scale to accurately predict 31P chemical shifts for novel species.
(A7)
Hersh, W. H.; Lam, S. T.; Moskovic, D. J.; Panagiotakis, A. J. “A Non-Karplus Effect: Evidence from Phosphorus Heterocycles and DFT Calculations of the Dependence of Vicinal Phosphorus-Hydrogen NMR Coupling Constants on Lone-Pair Conformation,” J. Org. Chem. 2012, 77, 4968-4979.
(B3)
Hersh, W. H. “False AA’X Spin-Spin Coupling Systems in 13C NMR: Examples Involving Phosphorus and a 20-Year-Old Mystery in Off-Resonance Decoupling,” J. Chem. Educ. 1997, 74, 1485-1489.
(B2)
Honeychuck, R. V.; Hersh, W. H. “Observation of Anion Spinning in the Dynamic 31P NMR Spectra of Fluorine-Bridged SbF6¯, BF4¯, and PF6¯ Adducts of R3P(CO)3(NO)W+. Implications for Barriers to Ionization and the Formation of Ion Pairs and Free Ions in Methylene Chloride and Hexane Solution,” J. Am. Chem. Soc. 1989, 111, 6056-6070.
(B1)
Honeychuck, R. V.; Hersh, W. H. “Observation of a Novel 31P NMR Cis-Influence Series: Implications for the Relative Basicity of PPh3 and PMe3 in Tungsten Carbonyl Complexes,” Inorg. Chem. 1987, 26, 1826-1828.
3. Electron-withdrawing phosphines and hydroformylation (papers A1, C1-3).
Typical triaryl or trialkyl phosphorus ligands in organometallic compounds are relatively electron-rich. We showed that N-sulfonyl phosphoramides such as TosL and diTosL and 1 above (C1, A1) are comparable to fluorinated phosphines in electron-withdrawing ability. Rhodium-catalyzed hydroformylation generally gives better results when relatively electron-poor phosphines are used, and we examined a variety of these ligands; results for diTosL are shown (C2, C3).
(C3)
Magee, M. P.; Li, H. Q.; Morgan, O.; Hersh, W. H. “Synthesis of Electron-withdrawing Butane- and Arenesulfonylamino Phosphines and use in Rhodium-catalyzed Hydroformylation,” Dalton Transactions 2003, 387-394.
(C2)
Magee, M. P.; Luo, W.; Hersh, W. H. “Electron-Withdrawing Phosphine Compounds in Hydroformylation Reactions. 1. Syntheses and Reactions Using Mono- and Bis(p-toluenesulfonylamino) Phosphines,” Organometallics 2002, 21, 362-372.
(A1)
Hersh, W. H.; Xu, P.; Simpson, C. K.; Wood, T.; Rheingold, A. L. “A Chiral N-Sulfonylphosphoramide: Synthesis and X-Ray Crystal Structure of a 1,3,2-oxazaphospholidin-5-one, a Trivalent Electron-Withdrawing Amino Acid-Derived Phosphorus Compound, and Synthesis of Its W(CO)5 Adduct.,” Inorg. Chem. 1998, 37, 384-385.
(C1)
Hersh, W. H.; Xu, P.; Wang, B.; Yom, J. W.; Simpson, C. K. “Synthesis of Tungsten Carbonyl and Nitrosyl Complexes of Monodentate and Chelating Aryl N-Sulfonylphosphoramides, the First Members of a New Class of Electron-Withdrawing Phosphine Ligand. Comparative IR and 13C and 31P NMR Study of Related Phosphorus Complexes,” Inorg. Chem. 1996, 35, 5453-5459.
4. Coordination of “noncoordinating” anions and Diels-Alder catalysis (papers B2, D1-5).
In the 1980’s we discovered a group of tungsten carbonyl nitrosyl Lewis acids that would reversibly bind the so-called “noncoordinating” anions SbF6–, PF6–, and BF4– via a bridging fluorine atom (D1, D4). X-ray structures showed that tungsten coordination stretched the Sb-F, P-F, or B-F bond but did not cleave it from the inorganic Lewis acids SbF5, PF5, and BF3 (see SbF6– adduct 1 with a Cy3P ligand below). 31P and 19F NMR spectra exhibited P-F coupling indicating anion coordination at low temperature, but at higher temperatures anion “spinning” via dissociation to tight ion pairs occurred followed by dissociation to free ions (D1, B2, D5).
The Me3P analogue of 1 is an efficient Diels-Alder catalyst, activating enones such as acrolein and methyl vinyl ketone by Lewis acid coordination of tungsten to the carbonyl oxygen as seen in the X-ray structure of 2 (D2) with acrolein coordinated to the tungsten cation. We examined chiral analogues such as 3 and 4 but have not achieved high yields with high enantioselectivity (D3).
(D5)
Hersh, W. H. “Synthesis and Characterization by 1H, 13C, and 19F NMR of (CH3CN)n(CO)4-n(NO)W(μ-F)BF3 and [(CH3CN)n+1(CO)4-n(NO)W][BF4] (n=0-2), Tungsten Mononitrosyl Carbonyl Cations with Labile Acetonitrile and [(μ-F)BF3]¯ Ligands,” Inorg. Chem. 1990, 29, 713-722.
(B2)
Honeychuck, R. V.; Hersh, W. H. “Observation of Anion Spinning in the Dynamic 31P NMR Spectra of Fluorine-Bridged SbF6¯, BF4¯, and PF6¯ Adducts of R3P(CO)3(NO)W+. Implications for Barriers to Ionization and the Formation of Ion Pairs and Free Ions in Methylene Chloride and Hexane Solution,” J. Am. Chem. Soc. 1989, 111, 6056-6070.
(D4)
Honeychuck, R. V.; Hersh, W. H. “Coordination of “Noncoordinating” Anions: Synthesis, Characterization, and X-ray Crystal Structures of Fluorine-Bridged [SbF6]¯, [BF4]¯, and [PF6]¯ Adducts of [R3P(CO)3(NO)W]+. An Unconventional Order of Anion Donor Strength,” Inorg. Chem. 1989, 28, 2869-2886.
(D3)
Bonnesen, P. V.; Puckett, C. L.; Honeychuck, R. V.; Hersh, W. H. “Catalysis of Diels-Alder Reactions by Low Oxidation State Transition Metal Lewis Acids: Fact and Fiction,” J. Am. Chem. Soc. 1989, 111, 6070-6081.
(D2)
Honeychuck, R. V.; Bonnesen, P. V.; Farahi, J.; Hersh, W. H. “Catalysis of Diene Polymerization and Diels-Alder Reactions by an Octahedral Tungsten Nitrosyl Lewis Acid. X-ray Crystal Structure of the η1-Acrolein Complex (cis-Me3P)(trans-NO)(CO)3W-O=C(H)-C(H)=CH2,” J. Org. Chem. 1987, 52, 5293-5296.
(D1)
Hersh, W. H. “Coordination of a “Noncoordinating” Anion: X-Ray Crystal Structure and 31P NMR Characterization of a Tungsten Nitrosyl Cation Ligated to SbF6¯,” J. Am. Chem. Soc. 1985, 107, 4599-4601.
5. Organometallic carbynes, alkylidenes, and acyls (papers E1-12).
Metal clusters have long been used to model the behavior of simple organic fragments on a metal surface in order to better understand complex reactions such as the Fisher-Tropsch conversion of CO and H2 to hydrocarbons. We found a general method for the synthesis of heterodinuclear alkoxycarbynes and discovered a novel oxygen to metal methyl migration reaction (E1, E6, E8, E9, E11), prepared a related μ-acyl (E2, E3), converted the carbynes to “difunctional clusters” containing both a carbyne and alkylidene ligand that couple to give a vinyl ligand (E4, E5), and examined the kinetics and crossover reactions of these processes (E7, E10, E12).
(E12)
Hersh, W. H.; Fong, R. H., “Crossover Studies of Methyl Migration from Oxygen to Iron in the Iron-Manganese Methoxycarbyne Complex Cp(CO)Fe(μ-COCH3)(μ-CO)Mn(CO)MeCp,” Organometallics 2005, 24, 4179-4189.
(E11)
Luo, W.; Fong, R. H.; Hersh, W. H. “Synthesis and Oxygen to Iron Methyl Migration Reaction of the Heterodinuclear Methoxycarbyne Complex Cp(CO)Fe(μ-COCH3)(μ-CO)Cr(CO)(η6-C6H6),” Organometallics 1997, 16, 4192-4199.
(E10)
Idmoumaz, H.; Lin, C. H.; Hersh, W. H. “Mechanism of Alkyl Migration from Oxygen to Metal in Iron-Manganese Ethoxycarbyne Complexes. Induction of Postcleavage Intermolecular Ethyl Exchange by Hydride Bridging of Mononuclear Iron Species,” Organometallics 1995, 14, 4051-4063.
(E9)
Wang, B.; Hersh, W. H.; Rheingold, A. L. “Synthesis and X-Ray Crystal Structure of the Heterodinuclear Iron-Chromium Carbonyl Nitrosyl Anion Complex [trans-(η5-MeCp) (CO) Fe(μ-CO)2Cr(NO)(η5-Cp)][Ph3PCH3], Conversion to a Heterodinuclear Methoxycarbyne Complex, and Kinetics of Thermally-Induced Oxygen to Iron Methyl Migration,” Organometallics 1993, 12, 1319-1330.
(E8)
Hersh, W. H. “Syntheses and Reactions of Heterodinuclear Alkoxycarbyne Complexes,” In Transition Metal Carbyne Complexes; F. R. Kreissl, Ed.; Nato ASI Series C: Mathematical and Physical Sciences, Vol. 392; Kluwer Academic Publishers: Dordrecht, 1993; pp 149-150.
(E7)
Hersh, W. H.; Hunte, F.; Siegel, S. “Kinetic Investigation of Phosphine Exchange in CpFe(CO)(L)CH3 (L = PPh3, PPh2Me) – Rate of Thermal Formation of the 16-Electron Species CpFe(CO)CH3,” Inorg. Chem. 1993, 32, 2968-2971.
(E6)
Fong, R. H.; Lin, C. H.; Idmoumaz, H.; Hersh, W. H. “Synthesis, Structure, Cis-Trans Isomerization, and Reactions of Heterodinuclear Iron Manganese Anion and Alkoxycarbyne Complexes. X-Ray Crystal Structure of cis-(η5-Cp)(CO)Fe(μ-COCH2CH3)(μ-CO)Mn(CO)(η5-MeCp),” Organometallics 1993, 12, 503-516.
(E5)
Fong, R. H.; Hersh, W. H. “Stereospecific Synthesis of (μ3-COCH3)(μ2-C(H)CH3)(Cp)(MeCp)Fe2Mn(CO)5, the First Carbyne-Ethylidene Cluster, and Intramolecular Hydrogen Migration to Give (μ2-C(H)OCH3)(μ3-CCH3)(Cp)(MeCp)Fe2Mn(CO)5, the First Carbene-Ethylidyne Cluster,” Organometallics 1988, 7, 794-796.
(E4)
Fong, R. H.; Hersh, W. H. “Synthesis and X-Ray Crystal Structure of (μ3-COCH3)(μ2-CH2)(Cp)(MeCp)Fe2Mn(CO)5, the First Carbyne-Methylene Cluster. Carbon-Carbon Coupling to Give a Methoxyvinyl Cluster,” J. Am. Chem. Soc. 1987, 109, 2843-2845.
(E3)
Bonnesen, P. V.; Yau, P. K. L.; Hersh, W. H. “Oxidative Syntheses of Cyclopentadienyl η2-Acyl Complexes and Stereospecific Conversion to an η2-Ylide Complex. X-Ray Crystal Structures of Cp(NO)(I)Mo(η2-C(O)-p-tol) and Cp(NO)(I)Mo(η2-C(O)(PMe3)-p-tol),” Organometallics 1987, 6, 1587-1590.
(E2)
Bonnesen, P. V.; Baker, A. T.; Hersh, W. H. “A New Metal-Metal Bond-Forming Reaction: Synthesis, Structure, and Mechanism of Formation of (η5-C5H5)(CO)Fe(μ-C(O)-p-tolyl)(μ-CO)Mo(NO)(η5-C5H5), a Rare π-Bound μ-Acyl Compound,” J. Am. Chem. Soc. 1986, 108, 8304-8305.
(E1)
Fong, R. H.; Hersh, W. H. “Synthesis and Reactions of the First Heterodinuclear Methoxycarbyne Complex (η5-C5H5)(CO)Fe(μ-COCH3)(μ-CO)Mn(CO)(η5-CH3C5H4),” Organometallics 1985, 4, 1468-1470.
6. X-ray structures (F1-3).
With the acquisition of a new X-ray diffractometer in 2011, we have carried out a series of structures on our own compounds and as collaborations with colleagues. These structures are just now starting to be published, with more than 10 structures currently in the pipeline.
(F3)
Chen, Y.; Liu, X.; Lee, M.; Huang, C.; Inoyatov, I.; Chen, Z.; Perl, A. C.; Hersh, W. H., “ICl-Induced Intramolecular Electrophilic Cyclization of 1-[4-Methoxy(1,1-biphenyl)2-yl]alkynones—A Facile Approach to Spiroconjugated Molecules,” Chemistry – A European Journal 2013, 19, 9795-9799.
(F2)
Dana, D.; Davalos, A. R.; Subramaniam, G.; Afzal, N.; Hersh, W. H.; Kumar, S., “A Base-Controlled Regioselective Synthesis of Allyl and Vinyl Phenyl Sulfones,” Tetrahedron Lett. 2013, 54, 2717-2721.
(F1)
She, Z.; Niu, D.; Chen, L.; Gunawan, M. A.; Shanja, X.; Hersh, W. H.; Chen, Y. “Synthesis of Trisubstituted Isoxazoles by Palladium(II)-Catalyzed Cascade Cyclization–Alkenylation of 2-Alkyn-1-one O-Methyl Oximes,” J. Org. Chem. 2012, 77, 3627-3633.
7. Miscellaneous and early papers.
(6) Kane, S.; Hersh, W. H. “Periplanar or Coplanar?,” J. Chem. Educ. 2000, 77, 1366.
(5) Hersh, W. H.; Bergman, R. G. “Kinetics and Mechanism of Decomposition of a Benzodicobaltacyclohexene: Reversible Dinuclear Elimination of o-Xylylene via a Dimetalla-Diels-Alder Reaction,” J. Am. Chem. Soc. 1983, 105, 5846-5859.
(4) Hersh, W. H.; Hollander, F. J.; Bergman, R. G. “Synthesis, Crystal and Molecular Structures, and Reactions of a Benzodicobaltacyclohexene, a Thermally-Derived Mononuclear o-Xylylene Complex, and an Unsymmetrical Phosphine-Derived Dinuclear Complex,” J. Am. Chem. Soc. 1983, 105, 5834-5846.
(3) Theopold, K. H.; Hersh, W. H.; Bergman, R. G. “Binuclear Metallacycles: Organometallic Ring Systems Containing Two Metal Centers,” Israel J. Chem. 1982, 22, 27-29.
(2) Hersh, W. H.; Bergman, R. G. “Synthesis and Reactions of a Dimetallacyclohexene. Thermal Conversion to an o-Xylylene Complex and Phosphine-Induced Conversion to Free o-Xylylene and a New Reactive Dinuclear Cobalt Complex,” J. Am. Chem. Soc. 1981, 103, 6992-6994.
(1) Katz, T. J.; Hersh, W. H. “The Stereochemistry of the Olefin Metathesis Reaction,” Tetrahedron Lett. 1977, 585-588.
Group resources: Major equipment includes a Vacuum/Atmospheres double station glove box equipped with a -35 °C freezer and a mini antechamber for fast pumping, vacuum lines, Biotage flash chromatography, departmental Bruker DPX400 MHz NMR equipped with a 1H/13C/19F/31P QNP probe, VT device, and 60-sample sample-changer and Bruker Avance500 MHz NMR, and a Bruker Smart Breeze X-ray diffractometer with Oxford cryoprobe.